Climate Change and Climate Variability
- Home
- Academics
- Departments & Units
- Atmospheric, Oceanic & Earth Sciences
- Research
- Climate Change and Climate Variability
Admission CTAs
Main navigation
Section Navigation: Research
Attribution and detection of climate change
Anthropogenic climate change will not only alter climate averages but also the probability distribution of weather events, raising the question of whether notable extreme events are caused – or at least made more probable – by climate change. For instance, in the South African metropolis of Cape Town, a severe drought brought millions of residents to the brink of “Day Zero,” the scenario in which reservoirs get so low that municipal water supplies run out and taps run dry. AOES scientists related the drought to long-term changes in the South Atlantic Subtropical Anticyclone (Burls et al., 2019).
On the other hand, an overlapping group of AOES scientists concluded that apparent changes in the relationship between El Niño-Southern Oscillation (ENSO) and the Indian Monsoon were a result of sampling variability rather than climate change (Cash et al., 2017). Similarly, Trenary et al. (2019) found that anthropogenically-forced changes in mid-twentieth century hurricane potential intensity were not detectable in the reanalysis products they examined.
Projections of changing climate variability
AOES Prof Stan has looked at the impact of climate change on the Tropical Atlantic vertical wind shear and its relationship with ENSO and changes in summer precipitation over the southeastern US in response to climate change. Additional model results help explain how and why the East Asian Summer monsoon will change in response to a warmer climate (Zhu et al., 2014; Zhu and Stan, 2015; Jin and Stan, 2019).
AOES Prof Dirmeyer and students have examined climate change simulations from many models, finding that climate sensitivity to variations in land surface conditions will expand (Dirmeyer et al. 2013, 2014a), meaning climate will become even more sensitive to anthropogenic land-use changes. Dirmeyer et al. (2014b) found increased water cycle extremes, both wetter and drier, that could specifically affect the regions where various staple crops can be grown. Maps of projected dates when climate change will push precipitation, runoff, and soil moisture beyond the envelope of 20th-century variability were presented by Dirmeyer et al. (2016).
Ocean Heat Uptake
Most of the excess heat absorbed by the Earth due to global warming enters the ocean. Ocean heat uptake helps determine the delay between a change in atmospheric greenhouse gas concentrations and the response of the Earth’s surface temperature. The degree of delay, currently poorly known, affects our estimates of how much the world will warm in the future.
A curious aspect of heat uptake is that the passive downward transport of heat by ocean currents and mixing is not the only mechanism for heat uptake. In addition, when ocean currents change due to global warming, they can redistribute already-existing temperature gradients within the ocean, effectively increasing or decreasing ocean heat uptake from the passive component.
Garuba and Klinger (2016) developed a way to diagnose the separate contributions of passive and redistributive heat uptake. They showed that, in idealized experiments, redistributive heat uptake due to weakening of the AMOC (see Ocean’s Role in Climate section above) was large in the Atlantic, but was exported to the Indo-Pacific sectors of the ocean. Contrary to expectations, Garuba and Klinger (2018) found that a weakening AMOC did not guarantee greater heat uptake. Due in part to the details of the surface flow in the northern North Atlantic, it is possible for redistribution to actually reduce, rather than increase, total heat uptake when the AMOC weakens. This implies that more care must be taken in projecting future heat uptake in the North Atlantic.
Past climates inform our future
As Tierney et al. (2020) say in the Science review article, “past climates inform our future”. Burls, a co-author on the Science paper, has been working under a CAREER grant to use ancient climates to help better understand the competing effects of anthropogenic emissions of CO2 and aerosols. The most recent period with CO2 levels as high as the present was the warm Pliocene Epoch (5.3-2.6 million years before present). Burls and colleagues at Yale University and elsewhere have been testing whether models can reproduce temperatures of the Pliocene, and even warmer climates during the Miocene and Eocene epochs.
Geological data indicates a weakened eastern equatorial Pacific “Cold Tongue” during the Early Pliocene, but climate models of the Pliocene do not typically reproduce the degree of weakening seen in the data. They also fail to fully capture polar-amplified warming reconstructed by proxy data. Burls and colleagues found that they could create a model climate with a weak Cold Tongue by changing cloud albedo. She has been investigating possible reasons for the modified albedo.
Land surface and climate change
Northern Europe is not a place we think of as experiencing water limitations. However, during the anomalously dry summer of 2018, Dirmeyer et al. (2021) found that soil moisture in Great Britain and large parts of Northern Europe dropped below a critical threshold where evapotranspiration could not cool the surface as efficiently, amplifying the extreme heat and drought conditions). This research was highlighted by the American Geophysical Union.
It has been commonplace to project climate change impacts from deforestation by replacing “space for time”, comparing deforested and forested areas in the current climate as a proxy for large-scale land-use change and its effects in the future. Chen and Dirmeyer (2020) developed a protocol for “fair” comparisons between contemporary observations and climate model simulations. The shift in agriculture into central North America between the middle 19th and late 20th centuries was found to affect afternoon precipitation and land-atmosphere interactions over much of North America (Chen and Dirmeyer 2017).
Climate model simulations suggest partial to total deforestation of the Amazon, driven by agricultural expansion, would have local and remote climate effects, affecting North America, Africa, and El Niño (Badger and Dirmeyer 2015, 2016a, 2016b). Meanwhile, the relative importance of land-use changes and increasing CO2 as a driver of temperature extremes was quantified by Chen and Dirmeyer (2019a), showing many regions have been cooled by changes in agriculture and land management, although the effect is becoming swamped by greenhouse gas warming. Impacts of irrigation on temperature extremes based on satellite observations and model simulations show that irrigation does not always result in cooling (Chen and Dirmeyer 2019b).
Climate Change: A Puzzle of Forced vs. Internal Variability
The Problem: When we observe climate variability, the question arises: Are these changes a result of natural climatic cycles (called internal), or are they driven by human activities or other external factors (called forced)? Disentangling forced and internal variability is crucial for understanding past climate changes and for accurately predicting future climate changes. Differentiating between these two sources of variability has challenged scientists for years.
The Traditional Approach: Traditionally, scientists have relied on climate models to discern forced variability. However, this raises a critical issue: Are these models accurately capturing forced variability? Doubts in this area cast a shadow on the reliability of conclusions drawn from such models.
An Alternative Approach: Dr. DelSole and his team are venturing down a different path. Their method turns the tables, focusing on understanding internal variability through climate models while making minimal assumptions about forced variability. This perspective offers an alternative look, free from the potential errors surrounding forced variability in climate models. This independent approach aligns closely with results from traditional methods, despite the vastly different assumptions, adding confidence to our understanding of climate variability.
Improving Earth System Models: The Key to More Accurate Climate Predictions
At the heart of predicting future climate changes lie Earth System Models (ESMs). These powerful tools are essentially virtual Earths, running on computers, solving complex laws of physics to predict the future. However, replicating Earth's climate system is no small feat. Some natural processes, like cloud formations, are too minuscule to be directly modeled in a global ESM. Yet, their impact is too significant to be ignored. This leads to a unique challenge: representing these small-scale processes through larger, resolvable ones, using empirical parameters fine-tuned against real-world observations. Selecting these parameters is a daunting task. Why? Because ESMs are not only computationally demanding but also require specifying hundreds of parameters. Identifying the optimal values for these parameters requires running ESMs for different parameter values and then choosing those that best match observations. Graduate student Nikki Lydeen has been working with Dr. Tim DelSole to develop a new approach for climate model parameter estimation that uses statistical and machine learning methods with a focus on computational efficiency.
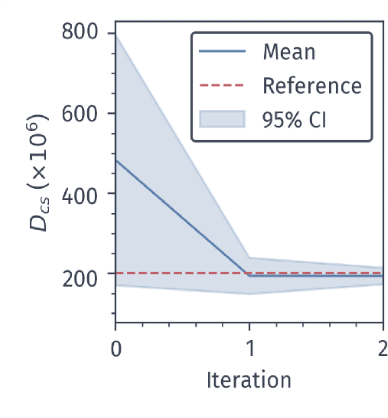
Student Opportunities
Student opportunities described below; contact the indicated faculty for details.
Undergraduate Research Opportunities
Undergraduates may work with faculty on the above research projects, or on projects developed independently by the student, supported by OSCAR or as independent research credits.
Graduate Research Opportunities
Graduates may work with faculty on the research projects, or independently with support from a GRA (when available), a College of Science GTA, or a successful fellowship.